Physicists Identify the Engine Powering Black Hole Energy Beams
Introduction
Paradoxically, black holes, those infamous swallowers of light and matter, also spew light and matter outward with unparalleled might and efficiency. They power thin beams of plasma called jets that extend thousands of light-years into space, forming glowing line segments seen all across the cosmos.
Physicists know why stuff goes in: Black holes have so much gravity that they trap even light, which cloaks them in spheres of invisibility. But why jets shoot out from the edges of many black holes has proved far harder to understand. “One of the biggest mysteries in the universe is how black holes launch jets,” said Sara Issaoun, an astrophysicist at Radboud University in the Netherlands.
Now, through the work of Issaoun and her colleagues on the black hole-observing Event Horizon Telescope (EHT) team, the mystery has started to unravel. Several weeks ago, the EHT released its second photo of a black hole — another view of the same fiery ring pitted by darkness seen in 2019. Both images show the glowing plasma around the supermassive black hole at the center of the galaxy M87, whose giant jet rises outside the frame. Unlike in the first photo, the ring in the new image has stripes, indicating that the light is strongly polarized.
Experts say the spiral pattern of the stripes results from a strong, orderly magnetic field around the M87 black hole, and that this represents the first significant empirical evidence in favor of a popular 44-year-old theory of jet launching, known as the Blandford-Znajek process.
Roger Blandford and Roman Znajek, young physicists at the University of Cambridge in 1977, argued that rotating supermassive black holes will twist ambient magnetic fields into a tight helix, and that this twisting will create a voltage that draws energy up and out of the hole and along the helix. This, they claimed, is the jet — and a big asterisk on the naive notion that nothing escapes black holes.
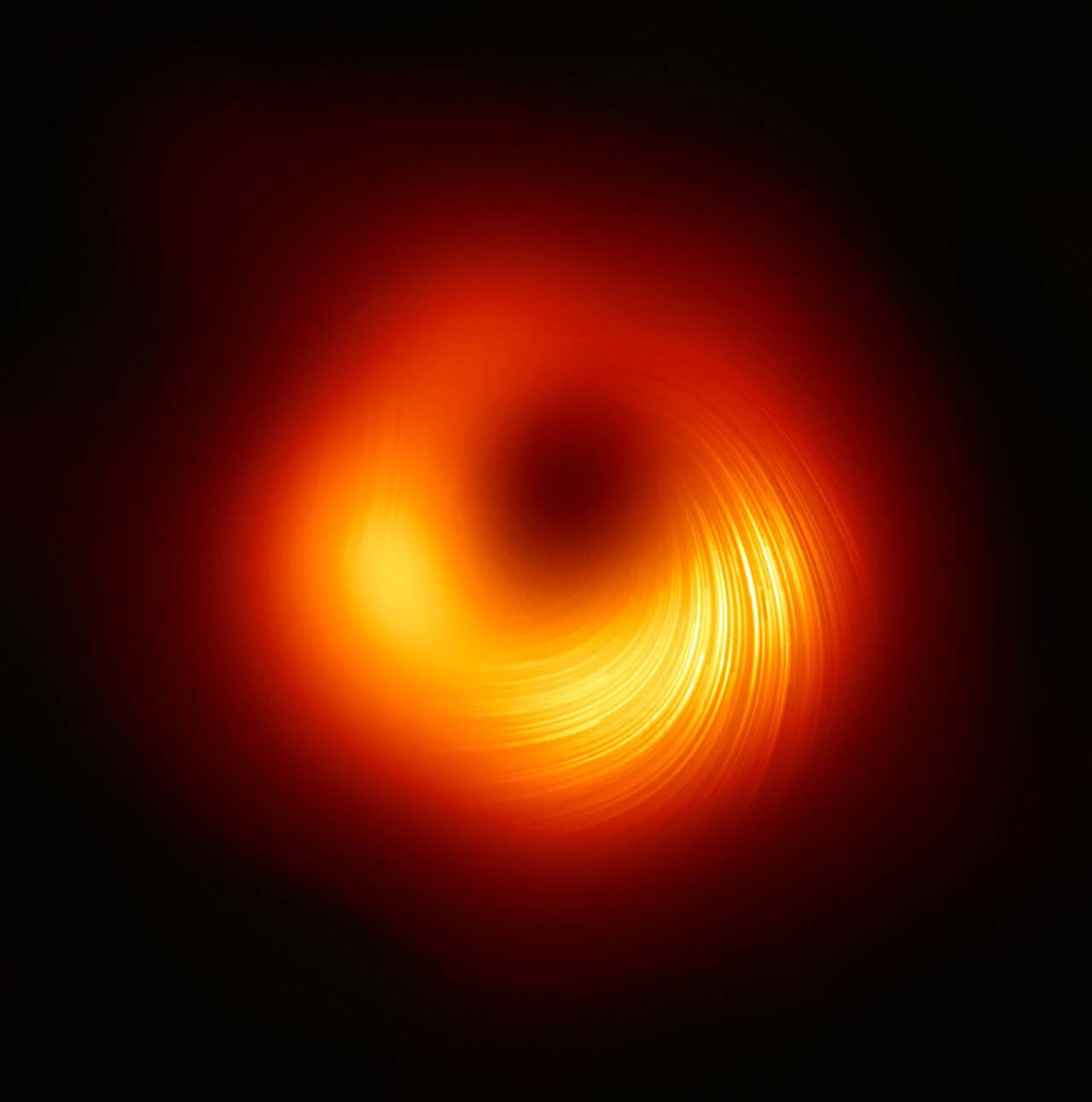
The stripes of polarized light around M87’s black hole reveal the strong magnetic fields at work.
EHT Collaboration
At the time, all ingredients of the process were speculative, but the new observations confirm the Blandford-Znajek idea. “What we see in our image is ordered polarization in a spiral shape,” said Issaoun, who was involved in analyzing the polarization measurements. “And the shape of the magnetic field is also spiral … which means it’s able to launch a jet.”
“I’m very chuffed,” Znajek, now a retired Cambridge city councilor, said by email.
Moreover, the new observations point to one of two rival versions of the Blandford-Znajek process that have been developed and explored in hundreds of computer simulations in recent decades, known as the MAD and SANE jet-launching scenarios. These competing ideas paint opposing pictures of a black hole’s milieu and, in particular, the origin and strength of its magnetic field.
SANE models, which assume weaker fields, were long considered more plausible. But the strongly polarized light in the Event Horizon Telescope’s new photo points to strong magnetic fields, and therefore to the MAD version of events. The new image “seems to heavily favor MAD models,” said Alexander Chen, a theoretical astrophysicist at the University of Colorado who is not part of EHT.
There’s more to figure out about black hole jets and their role in the cosmos. Opinions differ about several elements of the MAD scenario, and MAD leaves many questions unanswered that researchers are exploring in next-generation simulations. All the while, they remain in awe.
“The black hole in M87 is about the size of our solar system,” Issaoun said, yet it produces a 5,000-light-year-long current of white-hot plasma. That’s like the Statue of Liberty popping out of a marble. Some 3 trillion trillion trillion joules of energy flow up the jet each second — 500 trillion times more energy than the entire human population burns in a decade. “How could something so tiny be so powerful?”
The Wind-Up Helix
M87’s jet was the first ever discovered. Heber Curtis observed it in 1918 — “a curious straight ray” emanating from the center of a hazy patch of light that Curtis, a couple of years later, helped identify as a galaxy beyond our own. The theoretical possibility of black holes — snags in the otherwise smooth fabric of space-time — had been imagined only two years before, but it would take 60 years for Blandford and Znajek to connect black holes to jets.
They were a postdoc and a graduate student at Cambridge in the 1970s, when black holes were in the air. Stephen Hawking worked just down the hall. Roger Penrose was in London, doing the black hole theorizing that would eventually earn him the 2020 Nobel Prize in Physics. Astronomers were also starting to take black holes seriously, with observations suggesting that the X-ray source Cygnus X-1 was such an object.
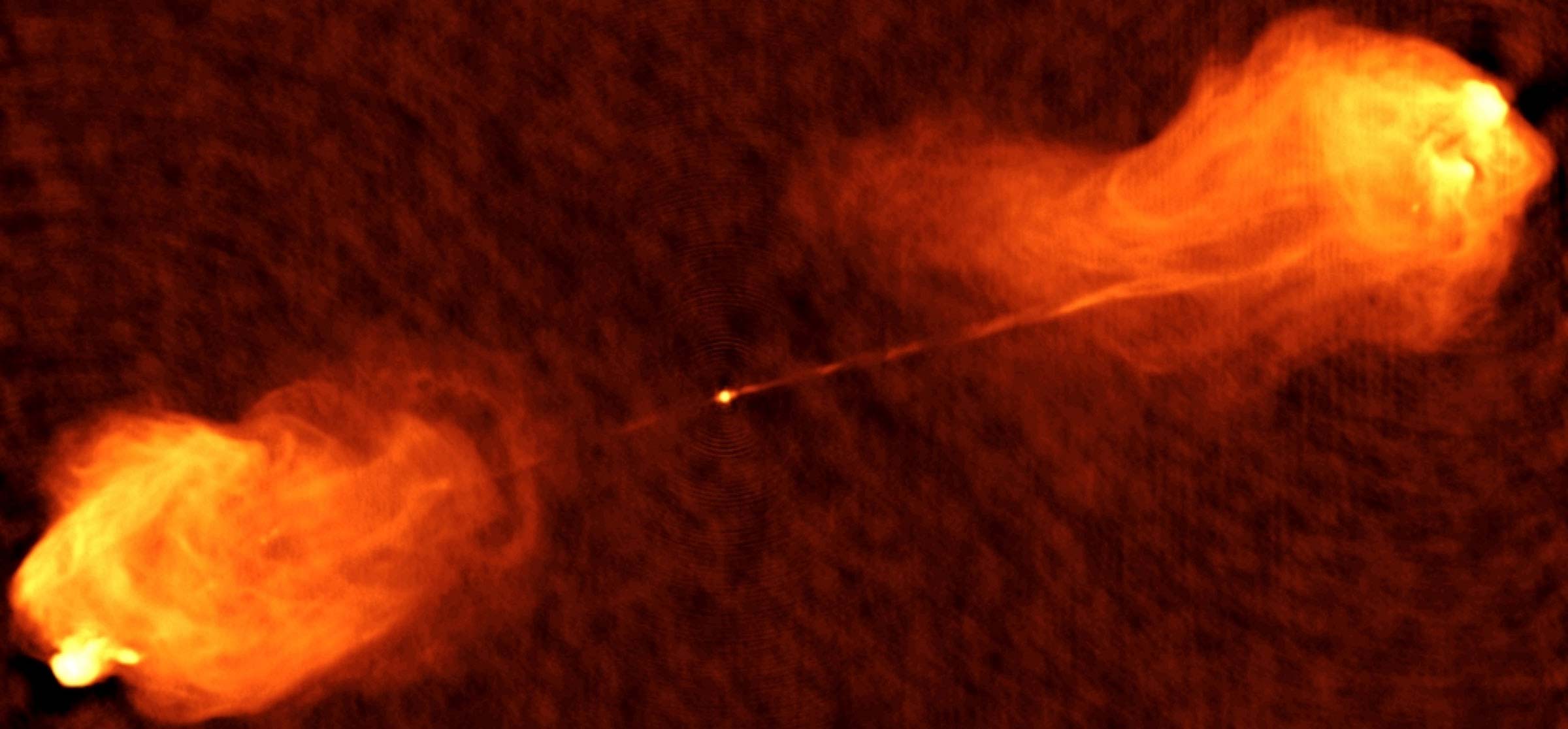
Jets emerging from the galaxy Cygnus A create massive interstellar blobs, visible here in radio waves.
NRAO/AUI/NSF
Blandford and Znajek were focused on the puzzling plethora of so-called double radio sources — huge, bright, radio-emitting blobs sitting on either side of distant galaxies. Theories abounded as to what these could be. Blandford and Znajek, immersed in their black hole sphere of influence, were early converts to the correct answer: The blobs spew from the ends of jets that shoot out in opposite directions from a big black hole in the galaxy’s center.
Astrophysicists would eventually confirm that supermassive black holes do, in fact, anchor galaxies, but at the time, Blandford and Znajek were speculating — not only about the presence of black holes, but also about their jet-generating capabilities. “The basic problem is you need a source of power,” said Blandford, who is now a professor at Stanford University, in a video call.
The mathematician Roy Kerr had solved the equations for a spinning black hole in 1963, showing that the hole, as it invisibly turns, drags the fabric of space-time around with it. Then Roger Penrose proved that spinning black holes can slow down, and that in doing so they transform their rotational energy into something else. “Both of us understood the Penrose process,” Blandford said, which proved that black holes “are not one-way membranes, as it were; you can extract the spin energy. We showed a way of doing that with electromagnetic fields.”
They knew that a big black hole at the center of a galaxy, because of its enormous gravity, will attract a large amount of interstellar gas. The gas will fall toward the black hole and rotate around it, forming an “accretion disk.” The gas will heat up, eventually getting so hot that atoms lose their electrons, creating a plasma that will carry magnetic fields.
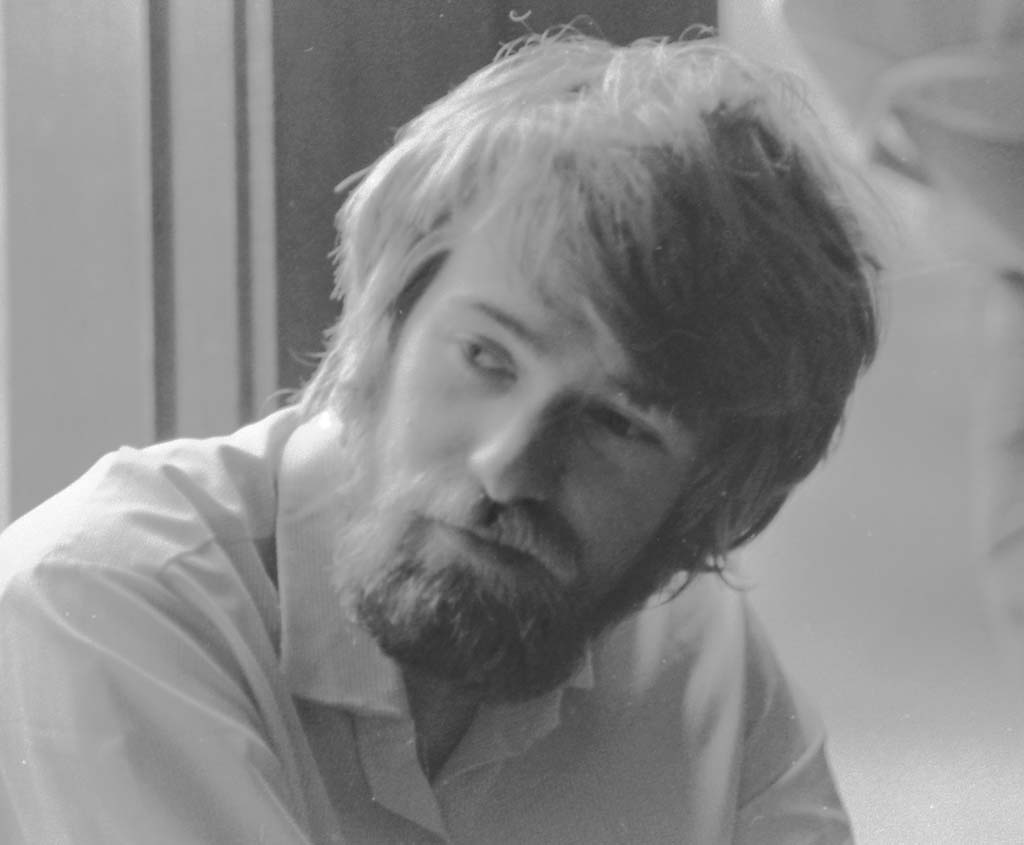
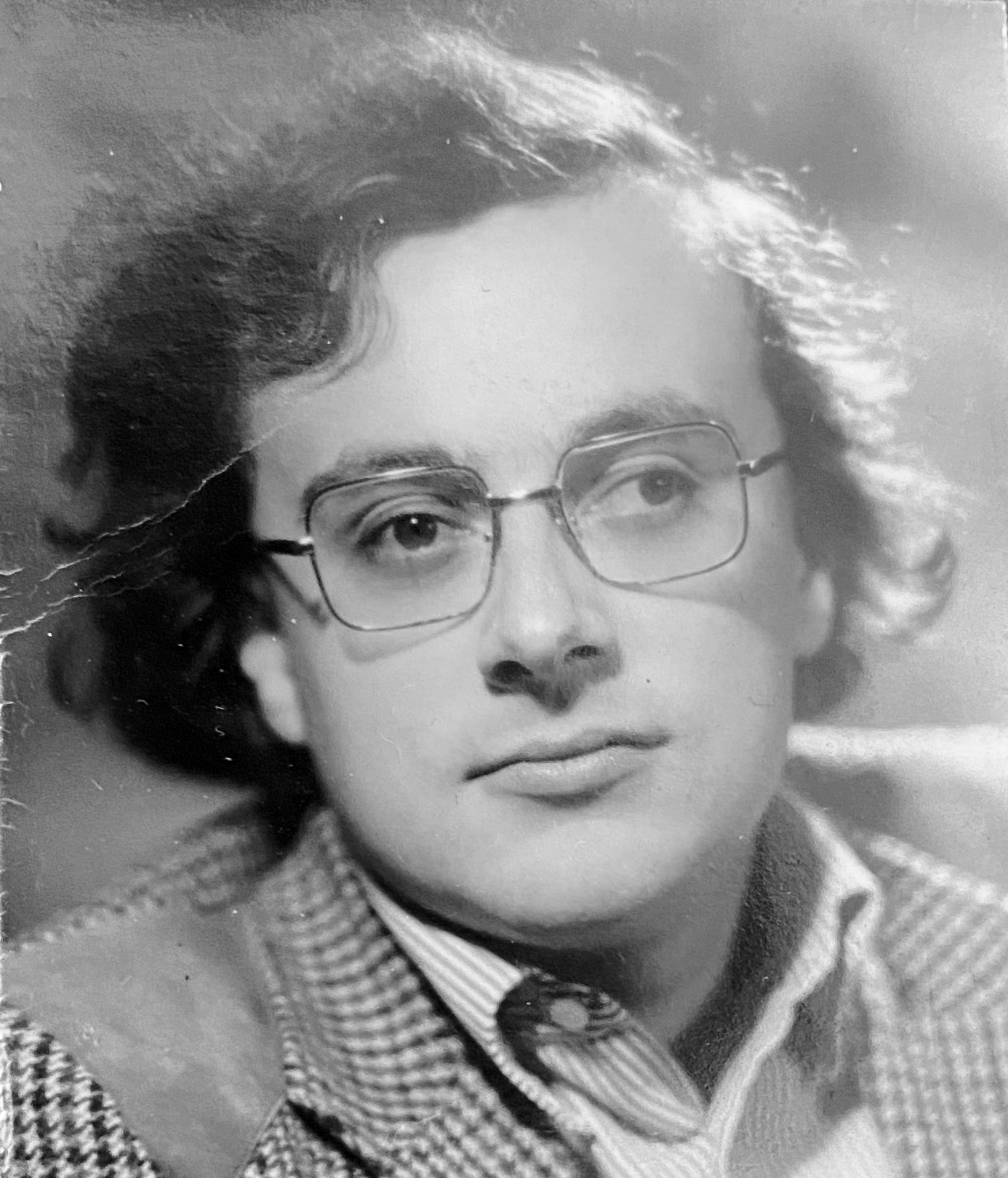
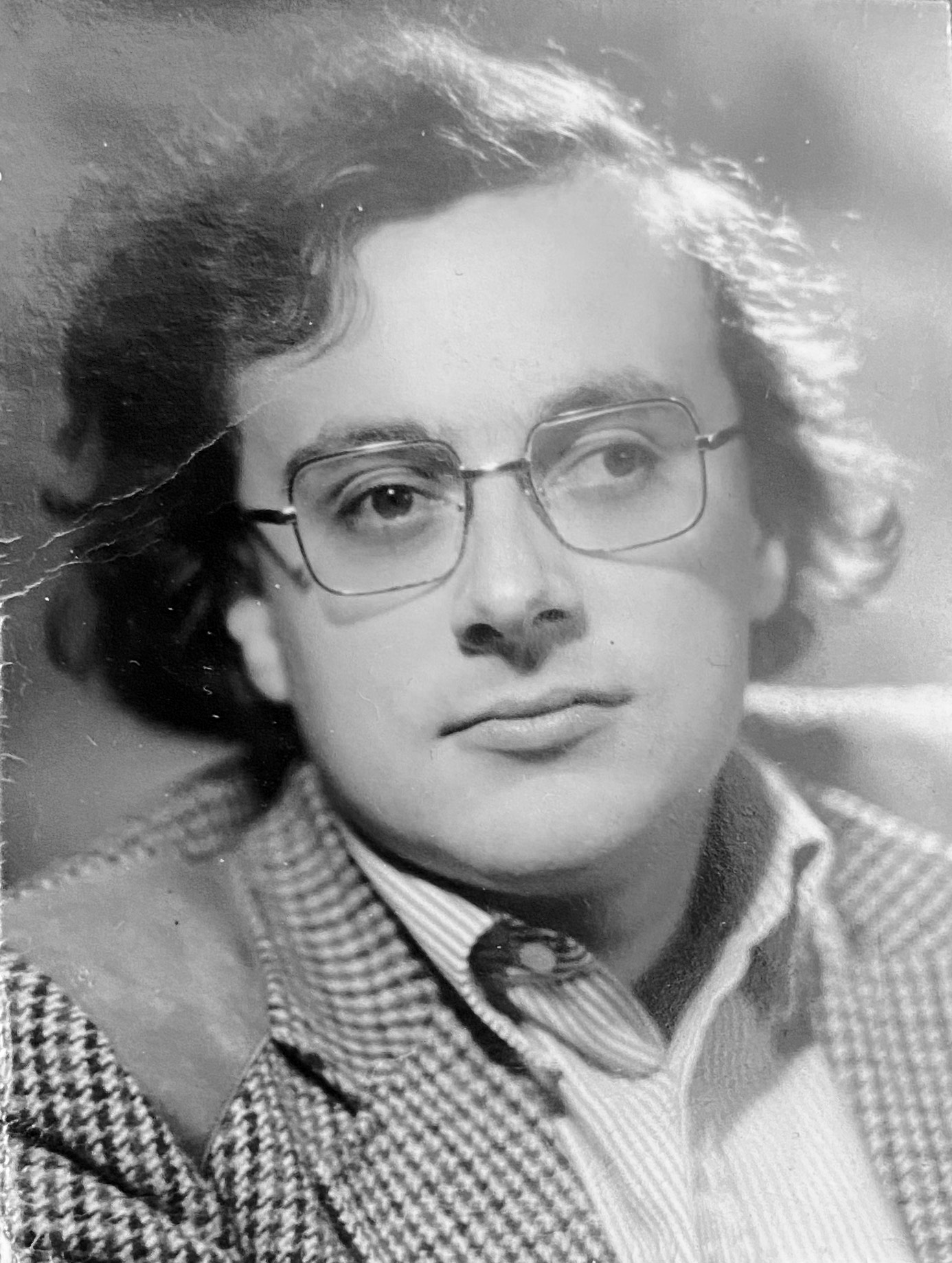
Roger Blandford (top) and Roman Znajek in photos from 1974 and 1977, respectively, when they were young researchers at the University of Cambridge.
Roger Blandford (left) and Roman Znajek in photos from 1974 and 1977, respectively, when they were young researchers at the University of Cambridge.
Courtesy of Roger Blandford; Edward Leigh / Provost and Scholars of King’s College via Courtesy of Roman Znajek
With Kerr’s equations in hand, Blandford and Znajek showed that when magnetic field lines from the accretion disk fall onto the spinning hole, the black hole’s rotation will wind the field lines into a helix oriented along the hole’s rotation axis. Magnetic fields in motion generate a voltage, so a current of electrons and positrons will start flowing through the helix away from the black hole in both directions. This is the jet.
In 1977, Blandford and Znajek’s jet-launching proposal seemed to work on paper. “Just proposing that this could be what’s happening was a huge step forward,” said Chen. But no one knew whether it was true.
Order and Chaos
As computing power improved in the ’80s and ’90s, people got the Blandford-Znajek process working in simulations. But every ingredient — the rotating black hole, the magnetic field, the light and matter in the accretion disk — was variable, and no one knew the right recipe.
A dichotomy developed in the 2000s between two classes of computer simulations: models in which the accretion disk runs the show, and models in which the magnetic field dominates.
At first, in the ’80s, researchers magnetized the accretion disks in their simulations just a little. In these models — later dubbed SANE, a backronym for “stable and normal evolution” — the plasma swirls around the black hole like water around a drain, and weak, randomly oriented, fluctuating magnetic field lines swirl with it. The turbulence of the field causes particles to collide and lose energy and angular momentum, allowing them to fall into the black hole rather than just orbiting around it. As the plasma falls in, it delivers weak magnetic field lines to the hole. Field lines gradually accumulate on it and are kept there by the influx of plasma. Eventually the black hole twists up this tangled field enough to launch a jet.
Samuel Velasco/Quanta Magazine; insets adapted from Michael O’Riordan et al.
Then in the late 1990s, researchers like Ramesh Narayan of Harvard University started cranking up the magnetic field strength around their simulated black holes just to see what would happen. These physicists found that when the field is strong enough, it becomes coherent rather than turbulent, and it controls the accretion disk rather than the other way around. Magnetic field lines form a literal force field around the black hole, acting as the sleeve for a jet while also preventing plasma from falling in the hole. Matter sometimes finds an opening, suddenly lurching through the magnetic barrier and slipping into the abyss. But for the most part, the disk is locked in place. These simulations became known as “magnetically arrested disk” or MAD models.
“For a long time people thought the SANE scenario is more natural,” said Andrew Chael, a physicist at Princeton University and a member of the EHT team who specializes in MAD models. Hot gas drifting toward the black hole from afar “is not falling in in a coherent way,” he said, so there was no reason to expect magnetism migrating inward with the gas to combine into something orderly and strong. “Five years ago, everyone was mostly doing SANE simulations,” Chael said.
But the Event Horizon Telescope’s new image of polarized light from around M87’s black hole points firmly in the MAD direction. Polarized light vibrates in a single plane, typically after reflecting off a surface or when emitted by charged particles whose paths are curving uniformly in a magnetic field. The spiral pattern in the photo conveys that the light’s plane of vibration rotates as you look at different places around the ring, exactly as expected if particles radiating the light are gyrating around magnetic field lines that themselves have a coherent spiral pattern.
A SANE situation, with feeble, turbulent fields, experts said, would create a much weaker polarization pattern. As it is, the field throughout the region is strong — as much as half as strong as a fridge magnet’s, Issaoun said, “but it’s also the size of a solar system.”
The Straight Path
To understand the origin of the curiously strong magnetic field around M87’s hole (and potentially all other supermassive black holes with jets), specialists must first crack the code of the stripy polarization pattern. “Right now people are trying very hard to infer through reverse engineering what the fields look like,” Chen said.
Meanwhile, other researchers have started to zoom out on the MAD situation; they’re simulating a larger region around the black hole in order to study how stars might generate the magnetic fields that migrate to the center. “Connecting this [magnetism] to larger scales is going to be a really big thing in the next few years,” Chael said.
Taking the opposite approach, a trio of young researchers — Sasha Philippov, Benoît Cerutti and Kyle Parfrey — are zooming in on the charged particles that shoot up the jet. Their detailed, particle-level simulations, which take millions of CPU hours, suggest that the particles mostly arise from photon collisions above the poles of the black hole, stealing energy from the hole as they shoot outward from there.
Studying the particles may be necessary for working out the jets’ overall structure and their effect on the galaxies and intergalactic space they pierce through. Many jets are thin and bright all along their length. “How does it shine? How do we see it?” Chen wonders. In the case of M87’s jet, “we see it very clearly in the sky. Sometimes it breaks into knots, and sometimes it’s continuous, but it’s remarkably straight and thin. Understanding that will definitely help us understand how it interacts with the galactic and intergalactic medium — how it transfers energy to the galaxy, for example.”
The EHT collaboration will continue to guide these efforts. By the end of this year they plan to publish a look at the black hole in our own galaxy’s center, a quiet beast called Sagittarius A* that may or may not have a faint jet. Follow-up observations of M87’s black hole will show how that hole’s environment varies with time, which will constrain how fast it’s spinning.
Even as the story of black hole jets gets written, the physicists’ sense of wonder about them seems, so far, undiminished. “You just have to look at these jets,” said Blandford, who continues to theorize about them. “They’re dead-straight things. They’re like lines on the sky, some of them. … It’s astonishing that nature is able to make these structures. Yeah, I am impressed.”
Correction: May 20, 2021
This article originally described Roy Kerr as an American physicist. While he spent part of his career in the U.S., and is best known for his work on black holes, he is in fact a mathematician from New Zealand. Quanta regrets the error.