The Molecular Bond That Helps Secure Your Memories
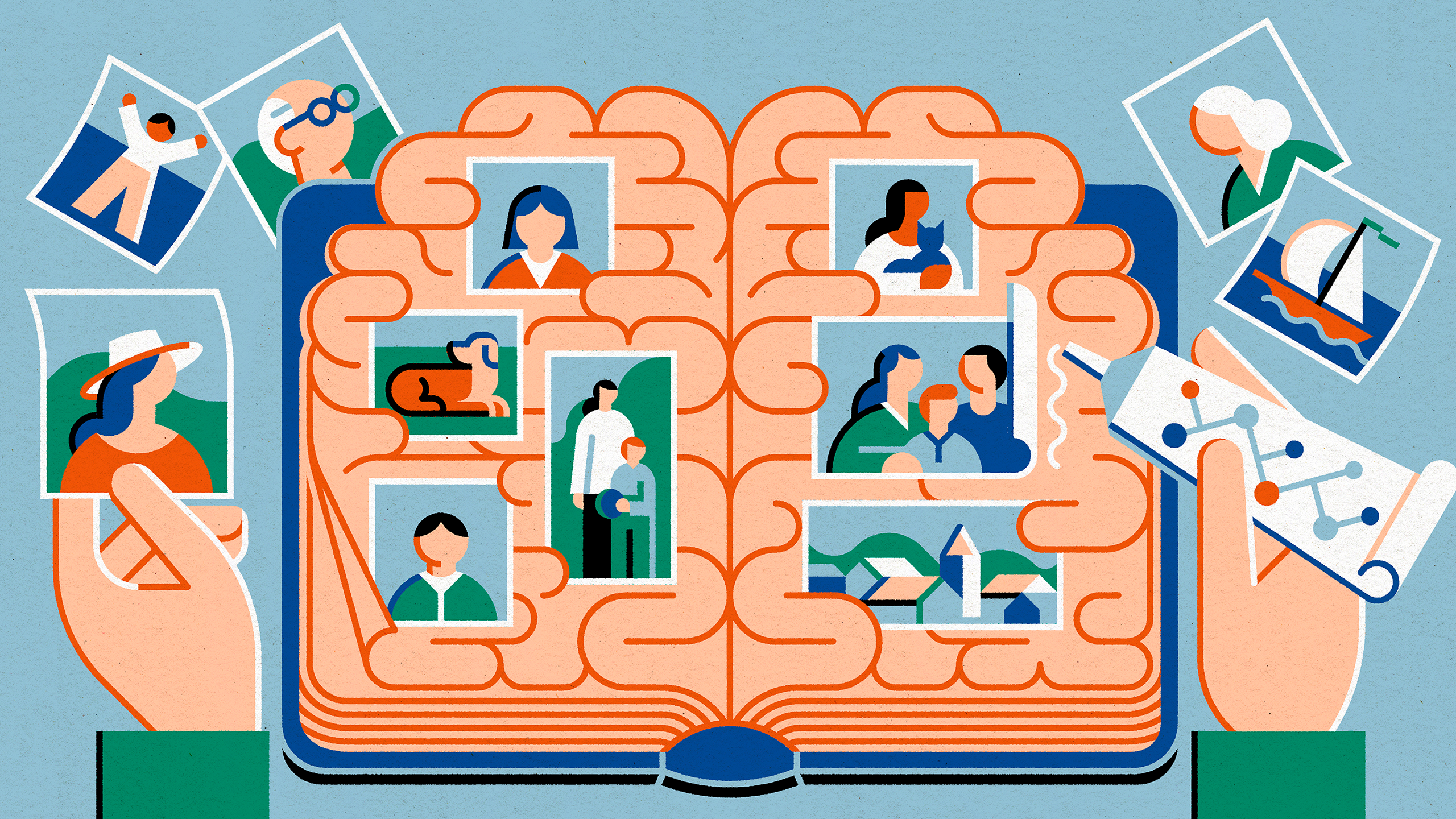
Carlos Arrojo for Quanta Magazine
Introduction
When Todd Sacktor was about to turn 3, his 4-year-old sister died of leukemia. “An empty bedroom next to mine. A swing set with two seats instead of one,” he said, recalling the lingering traces of her presence in the house. “There was this missing person — never spoken of — for which I had only one memory.” That memory, faint but enduring, was set in the downstairs den of their home. A young Sacktor asked his sister to read him a book, and she brushed him off: “Go ask your mother.” Sacktor glumly trudged up the stairs to the kitchen.
It’s remarkable that, more than 60 years later, Sacktor remembers this fleeting childhood moment at all. The astonishing nature of memory is that every recollection is a physical trace, imprinted into brain tissue by the molecular machinery of neurons. How the essence of a lived moment is encoded and later retrieved remains one of the central unanswered questions in neuroscience.
Sacktor became a neuroscientist in pursuit of an answer. At the State University of New York Downstate in Brooklyn, he studies the molecules involved in maintaining the neuronal connections underlying memory. The question that has always held his attention was first articulated in 1984 by the famed biologist Francis Crick: How can memories persist for years, even decades, when the body’s molecules degrade and are replaced in a matter of days, weeks or, at most, months?
In 2024, working alongside a team that included his longtime collaborator André Fenton, a neuroscientist at New York University, Sacktor offered a potential explanation in a paper published in Science Advances. The researchers discovered that a persistent bond between two proteins is associated with the strengthening of synapses, which are the connections between neurons. Synaptic strengthening is thought to be fundamental to memory formation. As these proteins degrade, new ones take their place in a connected molecular swap that maintains the bond’s integrity and, therefore, the memory.
The researchers present “a very convincing case” that “the interaction between these two molecules is needed for memory storage,” said Karl Peter Giese, a neurobiologist at King’s College London who was not involved with the work. The findings offer a compelling response to Crick’s dilemma, reconciling the discordant timescales to explain how ephemeral molecules maintain memories that last a lifetime.
Molecular Memory
Early in his career, Sacktor made a discovery that would shape the rest of his life. After studying under the molecular memory pioneer James Schwartz at Columbia University, he opened his own lab at SUNY Downstate to search for a molecule that might help explain how long-term memories persist.
The molecule he was looking for would be in the brain’s synapses. In 1949, the psychologist Donald Hebb proposed that repeatedly activating neurons strengthens the connections between them, or, as the neurobiologist Carla Shatz later put it: “Cells that fire together, wire together.” In the decades since, many studies have suggested that the stronger the connection between neurons that hold memories, the better the memories persist.
In the early 1990s, in a dish in his lab, Sacktor stimulated a slice of a rat’s hippocampus — a small region of the brain linked to memories of events and places, such as the interaction Sacktor had with his sister in the den — to activate neural pathways in a way that mimicked memory encoding and storage. Then he searched for any molecular changes that had taken place. Every time he repeated the experiment, he saw elevated levels of a certain protein within the synapses. “By the fourth time, I was like, this is it,” he said.
It was protein kinase M zeta, or PKMζ for short. As the rats’ hippocampal tissue was stimulated, synaptic connections strengthened and levels of PKMζ increased. By the time he published his findings in 1993, he was convinced that PKMζ was crucial for memory.
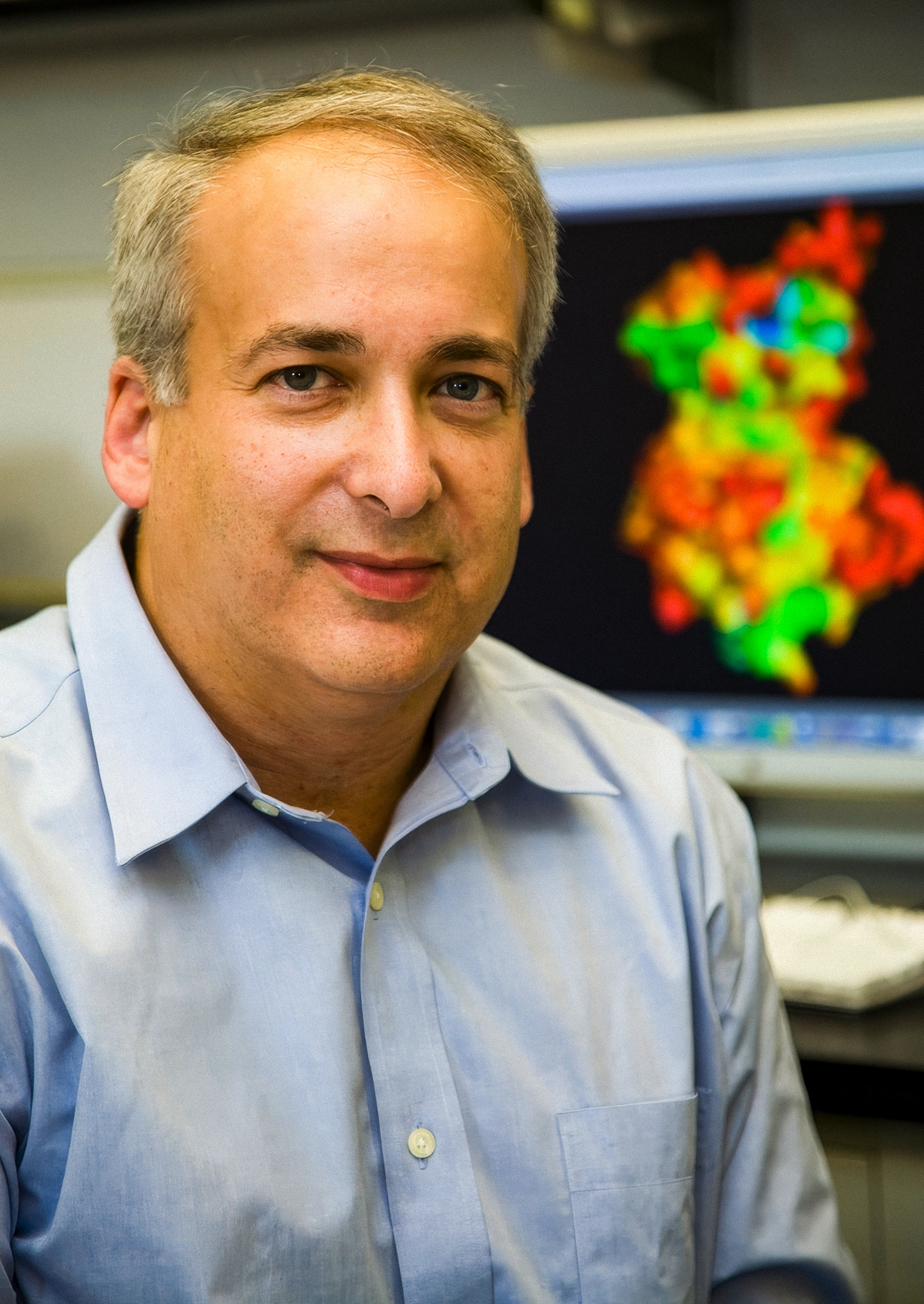
Todd Sacktor has devoted his career to pursuing the molecular nature of memory.
SUNY Downstate Health Sciences University
Over the next two decades, he would go on to build a body of work showing that PKMζ’s presence helps maintain memories long after their initial formation. When Sacktor blocked the molecule’s activity an hour after a memory was formed, he saw that synaptic strengthening was reversed. This discovery suggested that PKMζ was “necessary and sufficient” to preserve a memory over time, he wrote in Nature Neuroscience in 2002. In contrast, hundreds of other localized molecules impacted synaptic strengthening only if disrupted within a few minutes of a memory’s formation. It appeared to be a singular molecular key to long-term memory.
To test his hypothesis in live animals, he teamed up with Fenton, who worked at SUNY Downstate at the time and had experience training lab animals and running behavioral experiments. In 2006, the duo published their first paper showing that blocking PKMζ could erase rats’ memories a day or a month after they had formed. This suggested that the persistent activity of PKMζ is required to maintain a memory.
The paper was a bombshell. Sacktor and Fenton’s star protein PKMζ gained widespread attention, and labs around the world found that blocking it could erase various types of memories, including those related to fear and taste. PKMζ seemed like a sweeping explanation for how memories form and are maintained at the molecular level. But then their hypothesis lost momentum. Other researchers genetically engineered mice to lack PKMζ, and in 2013, two independent studies showed that these mice could still form memories. This cast doubt on the protein’s role and brought much of the ongoing research to a halt.
Sacktor and Fenton were undeterred. “We knew we had to figure it out,” Sacktor said. In 2016, they published a rebuttal, demonstrating that in the absence of PKMζ, mice recruit a backup mechanism, involving another molecule, to strengthen synapses.
The existence of a compensatory molecule wasn’t a surprise. “The biological system is not such that you lose one molecule and everything goes. That’s very rare,” Giese said. But identifying this compensatory molecule prompted a new question: How did it know where to go to replace PKMζ? It would take Sacktor and Fenton nearly another decade to find out.
The Maintenance Bond
A classic test of a molecule’s importance is to block it and see what breaks. Determined to pin down PKMζ’s role once and for all, Sacktor and Fenton set out to design a way to disrupt it more precisely than ever before. They developed a new molecule to inhibit the activity of PKMζ. It “worked beautifully,” Sacktor said. But it wasn’t clear how.
One day in 2020, Matteo Bernabo, a graduate student from a collaborating lab at McGill University, was presenting findings related to the PKMζ inhibitor when a clue emerged from the audience. “I suggested that it worked by blocking the PKMζ’s interaction with KIBRA,” recalled Wayne Sossin, a neuroscientist at McGill.
KIBRA is a scaffolding protein. Like an anchor, it holds other proteins in place inside a synapse. In the brain, it is abundant in regions associated with learning and memory. “It’s not a protein that a lot of people work on,” Sossin said, but there is considerable “independent evidence that KIBRA has something to do with memory” — and even that it is associated with PKMζ. Most research has focused on KIBRA’s role in cancer. “In the nervous system,” he said, “there are only three or four of us [studying it].” Sacktor and Fenton joined them.
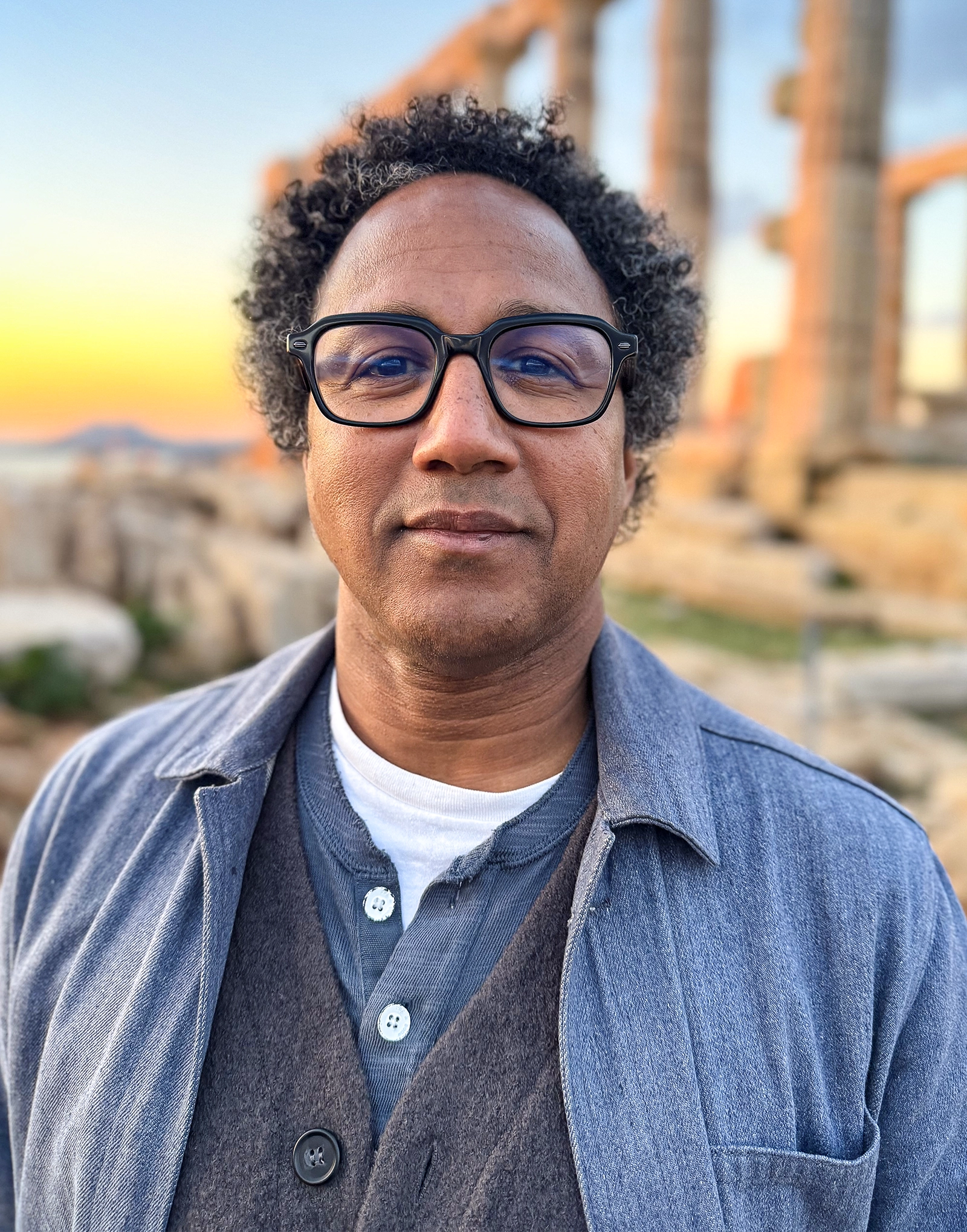
André Fenton and his team found that an interaction between two proteins is key to keeping memory intact over time.
Lisa Robinson
To find out if KIBRA and PKMζ work together in response to synaptic activity, the researchers used a technique that makes interacting proteins glow. When they applied electrical pulses to hippocampal slices, glowing dots of evidence appeared: Following bursts of synaptic activity that produced long-term synaptic strengthening, a multitude of KIBRA-PKMζ complexes formed, and they were persistent.
Then the team tested the bond during real memory formation by giving mice a drug to disrupt the formation of these complexes. They saw that the mice’s synaptic strength and task memory were lost — and that once the drug wore off, the erased memory did not return, but the mice could acquire and remember new memories once again.
But are the KIBRA-PKMζ complexes needed to maintain memory over the long term? To find out, the researchers disrupted the complex four weeks after a memory was formed. Doing so did indeed wipe out the memory. This suggested that the interaction between KIBRA and PKMζ is crucial not only for forming memories, but also for keeping them intact over time.
“It’s the persistent association between two proteins that maintains the memory, rather than a protein that lasts by itself for the lifetime of the memory,” said Panayiotis Tsokas, a researcher working with Sacktor and lead author on the new Science Advances paper.
The KIBRA and PKMζ proteins stabilize each other by forming a bond. That way, when a protein degrades and needs to be replaced, the other remains in place. The bond itself and its location at the specific synapses that were activated during learning are preserved, allowing a new partner to slot itself in, perpetuating the alliance over time. Individually, PKMζ and KIBRA don’t last a lifetime — but by binding to each other, they help ensure your memories might.
The discovery helps addresses the conundrum first identified by Crick, namely how memories persist despite the relatively short lifetimes of all biological molecules. “There had to be a very, very interesting answer, an elegant answer, for how this could come about,” Fenton said. “And that elegant answer is the KIBRA-PKMζ interacting story.”
This work also answers a question that researchers had put on the shelf. Sacktor’s earlier study showed that increasing levels of PKMζ strengthened synapses and memories. But how did the molecule know where to go within the neuron? “We figured, well, one day, maybe we’ll understand that,” Sacktor said. Now, the researchers think that KIBRA acts as a synaptic tag that guides PKMζ. If true, this would help explain how only the specific synapses involved in a particular physical memory trace are strengthened, when a neuron may have thousands of synapses that connect it to various other cells.
“These experiments very nicely show that KIBRA is necessary for maintaining the activity of PKMζ at the synapse,” said David Glanzman, a neurobiologist at the University of California, Los Angeles, who was not involved in the study. However, he cautioned that this doesn’t necessarily translate to maintaining memory because synaptic strengthening is not the only model for how memory works.
Glanzman’s own past research on sea slugs at first appeared to show that disrupting a molecule analogous to PKMζ erases memory. “Originally, I said it was erased,” Glanzman said, “but later experiments showed we could bring the memory back.” These findings prompted him to reconsider whether memory is truly stored as changes in the strength of synaptic connections. Glanzman, who has worked for 40 years under the synaptic model, is a recent proponent of an alternative view called the molecular-encoding model, which posits that molecules inside a neuron store memories.
While he has no doubt that synaptic strengthening follows memory formation, and that PKMζ plays a major role in this process, he remains unsure if the molecule also stores the memory itself. Still, Glanzman emphasized that this study addresses some of the challenges of the synaptic model, such as molecular turnover and synapse targeting, by “providing evidence that KIBRA and PKMζ form a complex that is synapse-specific and persists longer than either individual molecule.”
Although Sacktor and Fenton believe that this protein pair is fundamental to memory, they know that there may be other factors yet to be discovered that help memories persist. Just as PKMζ led them to KIBRA, the complex might lead them further still.